Numerical assessment of ceramic micro heat exchangers working with nanofluids by Taguchi optimization approach
- 1 Department of Mechanical Engineering, University of Mohaghegh Ardabili, Ardabil, Iran
- 2 SDU Life Cycle Engineering, Department of Green Technology, University of Southern Denmark, Campusvej 55, Odense M 5230, Denmark
Abstract
The rapid advancements in microsystems technology have necessitated the exploration of innovative materials for efficient thermal management in micro heat exchangers. This research delves into the performance evaluation of three advanced ceramics: ZrB2, BeO, and Si3N4 as alternative micro heat exchanger fabrication materials. The study systematically assessed the ceramics' interaction with Al2O3-nanofluids across diverse volume percentages and mass flow rates using the Taguchi optimization method. Beryllium oxide emerged as the superior material, registering warm outlet temperatures as low as 64.86 °C and cold outlet peaks at 31.68 °C. Sensitivity analyses further underscored the critical role of inlet temperature on outlet dynamics, with warm and cold outlets showing significances of ~72% and ~99%, respectively. Additionally, the research pinpointed 0.75 vol% as the optimal Al2O3-nanofluid content, yielding the most favorable performance metrics across the ceramics.
Downloads
References
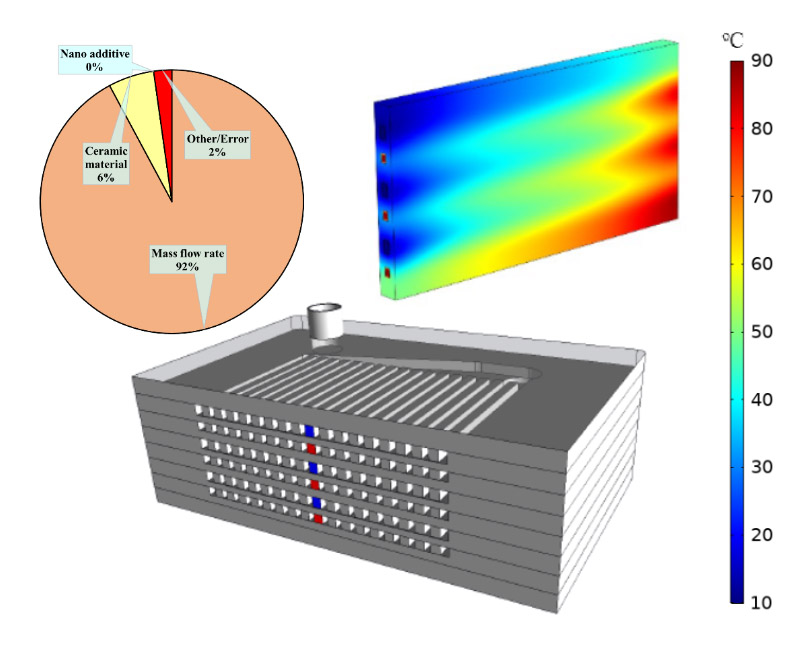
Copyright (c) 2023 Mohsen Naderi, Mohammad Vajdi, Farhad Sadegh Moghanlou, Hossein Nami

This work is licensed under a Creative Commons Attribution 4.0 International License.
Copyright
Authors are the copyright holders of their published papers in Synthesis and Sintering, which are simultaneously licensed under a Creative Commons Attribution 4.0 International License. The full details of the license are available at https://creativecommons.org/licenses/by/4.0/.
All papers published open access will be immediately and permanently free for everyone to read, download, copy, distribute, print, search, link to the full-text of papers, crawl them for indexing, pass them as data to software, or use them for any other lawful purpose without any registration obstacles or subscription fees.